Matthew M. Montemore, Ph.D.
Assistant Professor
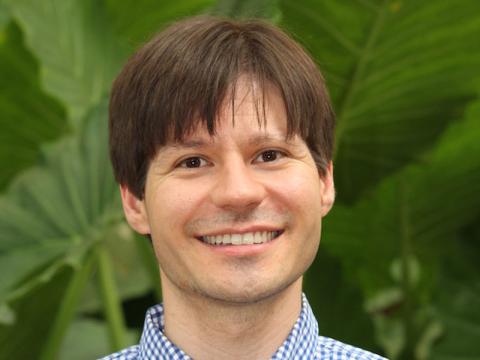
Office
323 Lindy Boggs Building
Department of Chemical and Biomolecular Engineering
Tulane University
New Orleans, LA 70118-5674
Education & Affiliations
Biography
As an undergraduate, Matthew M. Montemore majored in physics at Grinnell College. He became interested in research with applications in energy, and went on to earn his PhD in mechanical engineering at the University of Colorado Boulder. His research, performed in the Department of Chemical and Biological Engineering, focused on understanding and predicting adsorption on metal surfaces, with applications to catalysis and materials synthesis. For his postdoctoral work, he joined the School of Engineering and Applied Sciences and the Department of Chemistry and Chemical Biology at Harvard University. His research at Harvard has focused on using multiscale modelling, excited state dynamics, and machine learning to study the synthesis, structure, and chemical properties of nanostructured materials. He joined the Department of Chemical and Biomolecular Engineering at Tulane University as an assistant professor in January 2019.
Research Interests
Our group is interested in designing new materials for important energy applications. For example, we may want to develop a new catalyst for a fuel cell, a new absorber for a solar cell, or a new electrolyte for a battery. Our main focus is transition metal catalysts, which are used for energy-intensive industrial process and for clean energy technologies.
Using modern quantum chemical calculations, we can often calculate the properties of a material with enough accuracy to predict its performance. However, these calculations are computationally intensive, requiring hundreds or thousands of hours of CPU time, and we often wish to screen millions of possible materials. Therefore, the "brute force" approach to screening is too time and energy intensive to be practical.
To more effectively screen and design materials, we develop methods that allow much more efficient predictions of material properties. These can be based on our understanding of physics or chemistry, or can be based on finding patterns in data. In particular, we are interested in combining quantum chemical calculations, simple physical models, and modern machine learning techniques to create efficient, accurate, and robust models to predict material properties. After creating these models, we apply them to design new materials. The most promising candidates found using these efficient techniques are then more carefully using more computationally intensive methods. Finally, we collaborate with experimentalists to synthesize the materials and test our predictions.
Publications
M.M. Montemore, M.A. van Spronsen, R.J. Madix, C.M. Friend. O2 Activation by Metal Surfaces: Implications for Bonding and Reactivity on Heterogeneous Catalysts. Accepted to Chemical Reviews.
M.M. Montemore, A. Montessori, S. Succi, C. Barroo, G. Falcucci, D.C. Bell, E. Kaxiras. Effect of nanoscale flows on the surface structure of nanoporous catalysts. J. Chem. Phys. 146 (2017) 214703.
C. Barroo, M.M. Montemore, N. Janvelyan, B. Zugic, A. Akey, A.P. Magyar, J. Ye, E. Kaxiras, J. Biener, D.C. Bell. Chemistry-driven Kirkendall effect and the formulation of 3D nanoporosity. J. Phys. Chem. C 121 (2017) 5115.
M.M Montemore, E.D. Cubuk, J.E. Klobas, M. Schmid, R.J. Madix, C.M. Friend, E. Kaxiras. Controlling O coverage and stability by alloying Au and Ag. Phys. Chem. Chem. Phys. 18 (2016) 26844.
M.M. Montemore, O. Andreussi, and J.W. Medlin. Hydrocarbon Adsorption in an Aqeous Environment: A Computational Study of Alkyls on Cu(111). J. Chem. Phys. 145 (2016) 074702.
M.M. Montemore, R.J. Madix, E. Kaxiras. How does nanoporous gold dissociate molecular oxygen? J. Phys. Chem. C 120 (2016) 16636.
M L. Personick,M.M. Montemore, E. Kaxiras, R.J. Madix, J. Biener, C.M. Friend. Catalyst design for enhanced sustainability through fundamental surface chemistry. Philos. Trans. R. Soc. A 374 (2016) 20150077.
F. Hiebel, M.M. Montemore, E. Kaxiras, C.M. Friend. Direct visualization of quasi-ordered oxygen chain structures on Au(110)-(1x2) Surf. Sci. 650 (2015) 5.
J.W. Medlin and M.M. Montemore. Scaling rough heights. Nat. Chem. 7 (2015) 378.
T.D. Gould, M.M. Montemore, A. Lubers, L.D. Ellis, J. Falconer, J.W. Medlin. Enhanced Dry Reforming of Methane on Ni and Ni-Pt Catalysts Synthesized by Atomic Layer Deposition. Appl. Catal. A 492 (2015) 107.
M.M. Montemore and J.W. Medlin. A Unified Picture of Adsorption on Transition Metals Through Different Atoms. J. Am. Chem. Soc. 136 (2014) 9272.
M.M. Montemore and J.W. Medlin. Scaling Relations Between Adsorption Energies for Computational Screening and Design of Catalysts. Catal. Sci. & Technol. 4 (2014) 3748.
M.M. Montemore and J.W. Medlin. Predicting and Comparing C-M and O-M Bond of Strengths for Adsorption on Transition Metal Surfaces. J. Phys. Chem. C 118 (2014) 2666.
A.M. Robinson, M.M. Montemore, S.A. Tenney, P. Sutter, J.W. Medlin. Interactions of Hydrogen, CO, Oxygen, and Water with Molybdenum-Modified Pt(111). J. Phys. Chem. C 117 (2013) 26716.
M.M. Montemore and J.W. Medlin. Site-Specific Scaling Relations for Hydrocarbons on Hexagonal Transition Metal Surfaces. J. Phys. Chem. C 117 (2013) 20078.
M.B. Griffin, A.A. Rodriquez, M.M. Montemore, J.R. Monnier, C.T. Williams, J.W. Medlin. The selective oxidation of ethylene glycol and 1,2-propanediol on Au, Od, and Au-Pd bimetallic catalysts. J. Catal. 307 (2013) 111.
M.M. Montemore and J.W. Medlin. A Simple, Accurate Model for Alkyl Adsorption on Late Transition Metals. J. Phys. Chem. C 117 (2013) 2835.
M.M. Montemore and J.W. Medlin. A Density Functional Study of C1-C4 Alkyl Adsoprtion on Cu(111). J. Chem. Phys. 136 (2012) 204710.